Content Scribble
Reproduction, the genome and gene expression
The differences between sexual and asexual reproduction, the structure of DNA and its role in making proteins, mutations and their effects and how characteristics are inherited.
Advantages and disadvantages of sexual and asexual reproduction
Sexual reproduction involves the joining of two sex cells, or gametes during fertilisation. Organisms produced by sexual reproduction have two parents and are genetically similar to both but not identical to either.
The advantages of sexual reproduction:
- it produces variation in the offspring
- the species can adapt to new environments due to variation, which gives them a survival advantage
- a disease is less likely to affect all the individuals in a population
- humans can speed up natural selection through selective breeding, which can be used, for example, to increase food production.
The disadvatages of sexual reproduction:
- time and energy are needed to find a mate
- it is not possible for an isolated individual
Asexual reproduction only involves one parent so there is no joining of sex cells during fertilisation. Organisms produced by asexual reproduction are genetically identical to each other and their parent. They are clones.
The advantages of asexual reproduction include:
- the population can increase rapidly when the conditions are facourable
- only one parent is needed
- it is more time and energy efficient as you don’t need a mate
- it is faster than sexual reproduction.
The disadvatages of asexual reproduction include:
- it does not lead to variation in a population
- the species may only be suited to one habitat
- diseases may affect all the individuals in a population
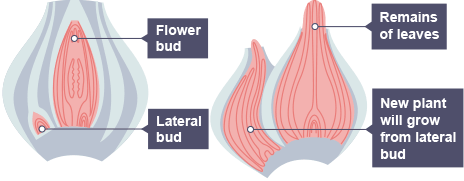
Meiosis
There are two types of cell division called mitosis and meiosis. Mitosis produces identical diploid body cells for growth and repair. Meiosis produces haploid nonidentical sex cells, or gametes. These fuse to form a diploid fertilised egg cell during fertilisation.
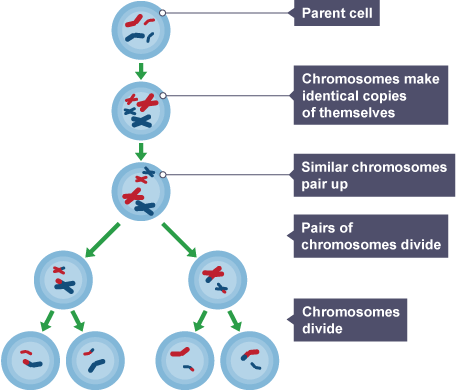
Meiosis produces sperm and egg cells in animals, and pollen and egg cells in plants.
From DNA to genomes
The genetic material in the nucleus of a cell is made up of a chemical called DNA. DNA is a polymer, made of many smaller units called nucleotides. A nucleotide is made of a sugar and a phosphate group, with one of four different bases, A, C, T or G, attached. The nucleotide join together, forming two strands. These, in turn, form a double helix structure. The double helix is help together by weak hydrogen bonding between complementary base pairs. Base A always pairs with T, and C always pairs with G forming a twisted ladder structure called a double helix. It carries the genetic code, which determines the characteristics of a living organism
Chromosomes
The cell’s nucleus contains chromosomes. These are long threads of DNA, which are made up of many genes.
Genes
A gene is a small section of DNA in a chromosome. Each gene codes for a particular sequence of amino acids in order to make a specific protein. It is the unit of heredity, and may be copied and passed on to the next generation.
The diagram shows the relationship between the cell, its nucleus, chromosomes in the nucleus, and genes.
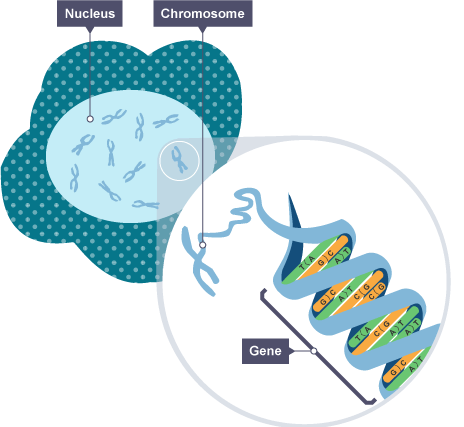
Genome
The genome is one copy of all an organism’s DNA. In humans this is all the DNA that makes up the 23 pairs of chromosomes found in all diploid body cells. That is all the cells except sex or gametes, which only have half of a person’s genome.
DNA structure and making proteins
The structure of DNA
James Watson and Francis Crick worked out the structure of DNA in 1953. By using data from other scientists (Rosalind Franklin and Maurice Wilkins) they were able to build a model of DNA. They X-ray crystallography data they used showed that DNA consists of two strands coiled into a double helix.
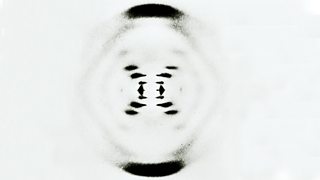
DNA is a polymer made from four different nucleotides. These are arranged in a repeating fashion. Each nucleotide consists of alternating sugar and phosphate sections with one of the four different bases attached to the sugar.

Base pairs
Each strand of DNA is made of chemicals called bases. Note that these different to bases to relation to acids and alkalis in chemistry. There are four different bases in DNA:
- thymine, T
- adenine, A
- guanine, G
- cytosine, C
There are chemical bonds between the two strands in DNA, formed by pairs of bases. They always pair up in a particular way, walled complementary base pairing:
- thymine pairs with adenine (T-A)
- guanine pairs with cytosine (G-C).
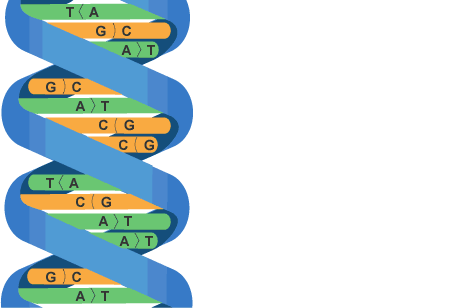
Genes are sections of the DNA. Each gene has the code for creating a specific protein. The sequence of bases in the gene controls which amino acids are joined in order to make a specific new protein (or enzyme) molecule. The proteins are then folded into their correct shaped to make them functional.
Protein synthesis
The DNA code for the protein remains in the nucleus, but a copy, called mRNA, moves from the nucleus to the ribosomes where proteins are synthesised in the cytoplasm. The protein produced depends on the template used, and if this sequence changes a different protein will be made.
Carrier molecules bring specific amino acids to add to the growing protein in the correct order. There are only about 20 different naturally-occurring amino acids.
Each protein molecule has hundreds, or even thousands, of amino acids joined together in a unique sequence. It is then folded in the correct unique shape. This is very important, as it allows the protein to do its job. Some proteins are enzymes, others are hormones and others form structures within the body, such as collagen. Each of these proteins needs a different shape.
Cells express their genes by converting the genetic message into protein. This process of protein synthesis occurs in two stages – transcription and translation.
Transcription
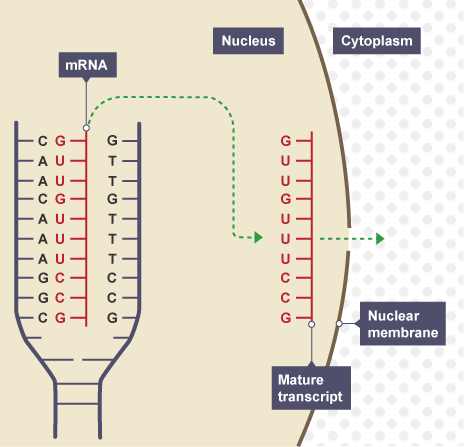
When a gene is to be expressed, the base sequence of DNA is copied or transcribed into mRNA (messenger RNA). This process takes place in the nucleus and occurs in a series of stages.
- The two strands of the DNA helix are unzipped by breaking of the weak Hydrogen bonds between base pairs. This unwinding of the helix is caused by an enzyme (helicase enzyme).
- The enzyme RNA polymerase attaches to the DNA in a non-coding region just before the gene.
- RNA polymerase moves along the DNA strand. Free RNA nucleotides form hydrogen bonds with the exposed DNA strand nucleotides by complementary base pairing to form a strand of mRNA:
- Note – RNA nucleotides contain the same bases as DNA, except that T is replaced by U. U base pairs with A.
- Because the opposite base bonds with the exposed DNA bases, the strand of mRNA is an opposite copy of the DNA strand (except that U replaces T). We call this a complementary copy.
- The newly formed strand of mRNA is now ready to leave the nucleus and travel to the ribosome.
Translation
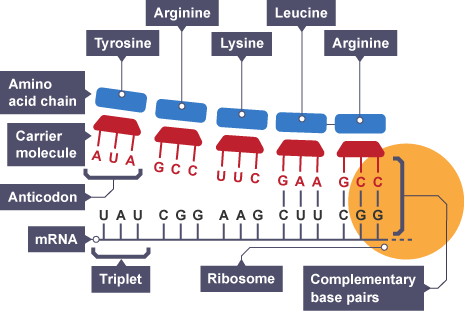
- The mRNA strand travels through the cytoplasm and attaches to the ribosome. The strand passes though the ribosome.
- For every three mRNA bases the ribosome lines up one complementary molecule of tRNA. We call every three bases a codon.
- tRNA molecules transport specific amino acids to the ribosome which they leave behind shortly after lining up opposite the DNA. Because there are three mRNA bases for each tRNA molecule, we call this the triplet code.
- Used tRNA molecules exit the ribosome and collect another specific amino acid.
- A chain of several hundred amino acids in the correct order according to the original DNA is then made. This is called a polypeptide.
After translation, the polypeptide is finally folded into the correct shape and becomes a protein. Peptide bonds form between the adjacent amino acids to finalise the structure.
Effect of genetic variants on phenotype
The structure of DNA is important in making specific proteins needed in biological processes.
Not all parts of the DNA code for proteins. In fact, it is believed that a human is made from only about 20,000 genes. This means that there are large parts of our DNA which don’t make proteins. We call these non-coding regions.
However, there are sections of non-coding DNA which can switch genes on and off. Not all the genes you need to survive are needed throughout your life. Some regions of these non-coding DNA are not as good as binding to RNA polymerase. This means the enzyme is less likely to bind and so less protein is produced. If less protein is produced this can affect the phenotype of the organism.
The diagram below shows DNA with certain genes switched on and others switched off:

Red boxes – genes switched on
White boxes – genes switched off
This diagram shows all of the genes are switched off:

In different cells around the body, certain genes will be switched on and others will be switched off. This will vary depending on which cells you examine.
Variation and the effect of mutation
Individuals in a population are usually similar to each other, but not identical. Some of the variation within a species is genetic, some is as a result of the environment they live in, and some is a combination of both.
Genetic causes of variation
Children generally look a little like their mother and their father, but are not identical to either. They inherit their features from each parent’s DNA.
Every sperm and egg cell contains half of the genetic information needed for an individual. Each sex cell is known as haploid, which has half the normal number of chromosomes. When the chromosomes fuse during fertilisation, a new cell is formed, which is known as a zygote. It has all the genetic information needed for an individual, which is known as diploid and has the full number of chromosomes.
Examples of genetic variation in humans include blood group, skin colour and natural eye colour.
Whether you have lobed or lobeless ears is due to genetic causes.
Sex is also an inherited variation – whether you are male or female is a result of genes you inherited from your parent.
Environmental causes of variation
Some examples of variation are not caused by the inheritance of genetics. Whether or not you have a scar or tattoo was not determined when the sperm fertilised the egg to begin your life. This variation is often caused by the environment we live in and so is called environmental variation.
Genetic and environmental causes of variation
Many kinds of variation are influenced by both environmental and genetic factors, because although our genes decide what characteristics we inherit, our environment affects how these inherited characteristics develop. For example:
- a person might inherit a tendency to be tall, but a poor diet during childhood will cause poor growth
- plants may have the potential for strong growth, but if they do not receive sufficient mineral resources from the soil, they may hardly grow at all
Identical twins are a good example of the interaction between inheritance and environment, because such twins are genetically the same. Any differences you may see between them – for example in personality, tastes and particular aptitudes – are due to differences in their experience or environment.
The causes and effects of mutations
Mutations are changes that can occur in genes. These changes are random and can be caused by background radiation and chemicals that we come into contact with, eg the chemicals in cigarette smoke. The change causes an alteration to the base sequence in the genetic code.
Sometimes these changes can be so severe that the cell dies, sometimes the cell can divide uncontrollably and become cancerous, and sometimes the changes are small and the cell survives. Occasionally, the changes may even be beneficial to us and produce new and useful characteristics.
Passing on mutations
If these changes occur in normal body cells, the changes are lost when we die. But if the changes occur in our sex cells such as sperm and ova, there is the possibility that the changes in the gene will be passed onto the next generation.
It is when these changes are passed on to the next generation that natural selection can either ensure that they are selected if they are useful, or disappear from the gene pool if they are not.
0 Comments